Article by Colin Fricker1, Dilidaer Yusufu2 and Andrew Mills2
Oculer Ltd, Ballina, Ireland1 and School of Chemistry and Chemical Engineering, Queens University, Belfast, UK2
Why use O2 sensors?
O2 sensors are widely used in many areas of biology and they have been applied for monitoring the activity and growth of bacteria in the environment. There are a small number of reports of their use for the specific detection of microbial growth in the laboratory. A combination of appropriate culture media, temperature and an immobilised O2 depletion sensor can consistently and accurately detect the growth of microorganisms including bacteria, yeast and fungi. As the organisms grow, they deplete the level of O2 in the culture medium and, at a certain point in time, this depletion impacts the sensor resulting in a very rapid increase in the lifetime of the dye, which can be detected by an appropriate instrument.
Using an appropriate O2 sensor together with a suitable immobilising polymer results in a detection system which has a long shelf life, and is heat resistant, not impacted by pH or ionic strength of the medium. It is important to note that because the polymer that is used to immobilise the sensor is impermeable to most molecules other than gases, the sensor is not impacted by different food matrices or ionic species. The lack of impact of pH means that media can be buffered to a pH >7.0 to allow faster growth and recovery of certain organisms, something which is detrimental to the detection of carbon dioxide as, under such pH conditions, CO2 is initially converted to bicarbonate, resulting in longer detection times.
The theory behind O2 sensors
Almost all commercial O2 optical indicators are based on the ability of O2 to quench (i.e. suppress) the luminescence exhibited by a dye, D. The range of dyes for this purpose are limited and most contain platinum or ruthenium. D will luminesce when it is exposed to light it absorbs; the excitation light. The wavelengths, i.e. colours, of the excitation and resulting luminescence light are usually very different, which makes monitoring the latter easy. If the excitation light is delivered as a constant stream to the indicator, then it will luminesce continuously.
However, if, as in O2 sensing, it is delivered in the form of a very short pulse, i.e. a flash, the luminescence intensity will instantly peak and then decay to zero. In practice, the time it takes for this decay to end is difficult to determine and so, instead, the time is measured for the peak luminescence intensity to decrease by a defined fraction. In photochemistry in general and O2 sensor technology specifically, this fraction is always the reciprocal of the natural number, e, (2.71828) and so the fraction is 0.368. The time is referred to as the luminescence lifetime, Τ, of the dye.
For all luminescent dyes, the value for Τ in the absence of O2, i.e., Τo, is characteristic of the dye, and so is different for every dye. For O2-sensitive dyes, Τo is typically 40-100 ms and Τ decreases with the increasing partial pressure of O2, PO2, according to the Stern-Volmer equation,
Τo/Τ = 1 + KSVPO2
where KSV is the Stern-Volmer constant, which depends both on Τo and how quickly the O2 moves to the dye and quenches its luminescence.
In most commercial O2 optical indicators, the luminescent dye is embedded in an O2-permeable plastic, to form a coloured ‘dot’ – often green due to the usual Pt porphyrin dye used in this work. The sensor dot is usually deposited on the wall of a transparent bottle or sample tube, that contains the medium, aqueous or gaseous, under test. The lifetime of the dye in the dot is then monitored using a sensor probe pressed against the sample tube, opposite the O2 ‘dot’, as illustrated in Figure 1. The sensor probe provides the excitation light and collects the luminscence light and measures its lifetime.
When applied in microbiology to determine the log (cfu) in a test sample, a growth medium is placed in a vial and inoculated with the sample under investigation. As the bacteria grow in the medium, the level of dissolved O2 will decrease from its initial value associated with an air-saturated solution, i.e. 0.21 atm, to zero and so the measured value of Τ will increase from its value in air saturated solution to Τo. A typical set of Τ vs incubation time profiles for a range of different cfu inoculation values is illustrated in Figure 1. These profiles can be used to create a simple, straight line calibration graph relating log (cfu) to the time taken to reach a set value of Τ, i.e. a specific level of O2. This calibration graph can then be used to determine the value of the log (cfu) in a test sample for the bacteria.
Figure 1
Simplicity of testing
The use of systems which allow the direct detection of changes in culture media due to microbial growth allow for extremely simple detection in a far shorter period of time. Thus, typically, the sensor is immobilised in a culture vessel and the culture media added. The sample under test is then added directly to the vessel and incubated in a combined incubator/reader for the duration of the test. The sensor is interrogated at frequent intervals and when significant O2 depletion is detected, a change in reading is obtained, alerting the user that the sample contains microorganisms of interest. Because there is no need to wait for colonies to form, as required in the traditional methods used to determine microbial load, the results are available quickly and the higher the microbial load, the faster a positive signal is obtained.
The Oculer Rapid 930 Series
The Oculer Rapid 930 Series, marketed by Oculer Ltd is a self-contained benchtop instrument incorporating an incubator and luminescence detector, the use of which requires no specific microbiological expertise. Sample vials, containing appropriate culture media are supplied after gamma irradiation. There are two versions of the instrument suitable for incubation of 48 x 2 ml vials or 24 x 15 ml vials. If samples are incubated offline, the total number of samples that can be run increases to 432 and 216 respectively. When used in quantitative mode the Oculer instrument calculates the concentration of target bacteria and the results are expressed as colony forming units.
The system has been used for a wide variety of matrices including dairy and non-dairy beverages, carbonated beverages, yoghurt, process water, fruit juice and a plethora of other liquid and semi-solid matrices. Assays are available for total microbial count, yeast and mould, total coliforms, E.coli, Alicyclobacillus spp. and commercial sterility. There is also a kit available for environmental monitoring of surfaces. The sample size used can be up to 10 ml depending on the assay. Most assays are complete within 24 hours, the time for a yeast and mould assay is reduced from 5-7 days to 48 hours and for Alicyclobacillus the assay time is a maximum of 72 hours.
Use of Oculer Rapid 930 Series for commercial sterility testing
The Oculer system is ideally suited for the determination of commercial sterility. Using a large sample size (10 ml) together with a small amount of a concentrated growth medium allows the detection of growth of only those organisms that can grow in the actual product. By using a minimal dilution this ensures that the pH and concentration of naturally occurring microbial inhibitors is close to that of the original product, preventing the growth of organisms that are not of concern as they would not be capable of growth in the finished product. This greatly enhances the reliability of the test.
A recent study using plant-based flavoured milks and other non-alcoholic beverages (496 samples) demonstrated that the system was more sensitive and faster when compared to standard plating procedures. Samples of finished product were inoculated with low numbers of potential contaminants (1-10 cfu/100 ml) and incubated for seven days. Additional samples were prepared by dilution to extinction, before incubating for seven days. After incubation, aliquots were removed and analysed by spread plating, pour plating and by the Oculer Rapid 930 Series.
The Oculer system detected growth in more samples than the traditional pour plate technique and yielded results within 48 hours (in fact the longest period of incubation to register a positive result was 29 hours and 11 minutes). Not surprisingly pour plates detected more positive samples than spread plates. The system was used to examine 10 ml of sample, pour plates 1 ml and spread plates 0.1 ml. It is clear that in many cases, even after seven days incubation that the numbers of organisms present are relatively low and this is demonstrated by the differences in detection using different sample volumes.
Use of a large (10 ml) sample increases sensitivity and significantly reduces the overall time to detect contamination. Other methods of detecting growth after the initial incubation which use even smaller sample sizes, such as ATP, while providing a rapid result will miss a substantial number of positive samples that would be detected by the Oculer Rapid 930 Series. Use of the system offers substantial advantages for commercial sterility testing, both in terms of speed and sensitivity.
For more information on the Oculer Rapid 930 Series use the Request Information button below.
About the Authors:
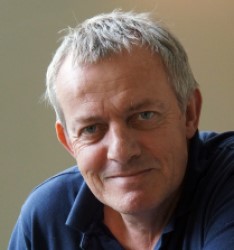
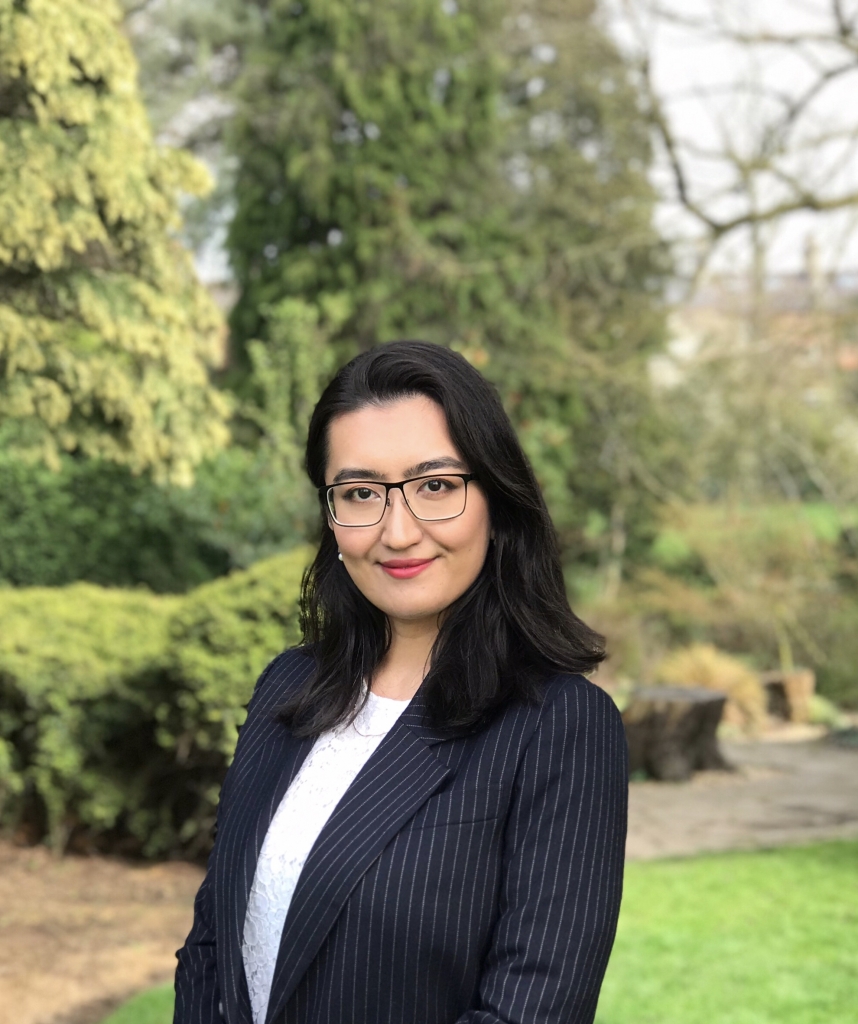
Andrew Mills is Professor of Materials Chemistry at the School of Chemistry and Chemical Engineering, Queen’s University, Belfast. His research interests include: dye and semiconductor photochemistry, redox catalysis, solar energy conversion and colour and fluorescence based indicators. He is the 2019 recipient of the RSC Materials for Industry - Derek Birchall Award, for his pioneering work on smart – optical sensor - inks, pigments and extruded plastics technologies.