PCR for the Food Microbiology Laboratory
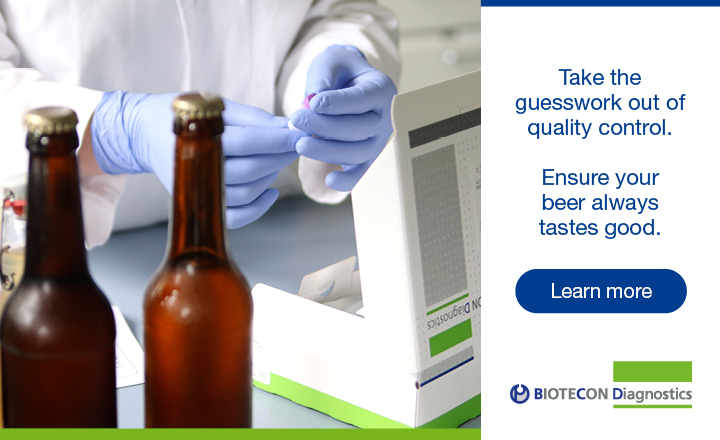
- Rapid and automated – results usually available within 12 to 26 hours
- High degree of sensitivity – able to detect one colony forming unit in a 25g sample
- Precise and accurate results from very specific genetics-based detection
- Flexible – assays for more than one pathogen can be run simultaneously
The most remarkable thing about the typical food microbiology laboratory is how little it has changed in the last 20 years. Time saving devices may have been introduced in that time, but the underlying culture-based technology employed would be familiar to laboratory staff in the 1980s.
This is all the more surprising when one is aware that the recent development of molecular biology has provided a whole new range of tools that have re-vitalised many branches of microbiology. Now food microbiologists too are benefiting from this revolution as some of the key molecular biology tools begin to appear in their laboratories.
Foremost among these tools are PCR (polymerase chain reaction)–based techniques that target the genetics of microorganisms, rather than their phenotypic characteristics.
What is PCR? PCR is a method for synthesising multiple copies of (amplifying) a specific piece of DNA. For PCR to take place four basic components are required:
- A DNA template containing the target sequence that is to be amplified. For pathogen detection this sequence must be highly specific to the organism concerned, often a single gene, such as a virulence gene.
- Primers – a pair of short single-stranded DNA sections, which are exactly complementary for specific parts of the target sequence.
- A heat-stable DNA-polymerase enzyme, usually Taq polymerase from a thermophilic bacterium, which catalyses the reaction.
- Free nucleotides that are used as the building blocks for multiple copies of the DNA template.
The first stage in the PCR process is to raise the temperature to about 90-95oC. This causes the double stranded DNA to denature, or melt, into single strands.
The temperature is then reduced to about 50-65oC to allow the two primers to bind, or anneal, at specific points on the single-stranded DNA of the target sequence.
Finally, the temperature is raised to 70-74oC and the DNA-polymerase enzyme catalyses the duplication of the target sequence, starting at the annealed primers on each single strand, in a process known as extension. This results in two double-stranded DNA fragments that are identical copies of the original target sequence.
The temperature cycling process is then repeated a number of times, typically 30-40, creating a theoretical doubling of the number of copies of the target sequence at each cycle. This gives an exponential increase in target DNA concentration and produces sufficient DNA for reliable detection from a single target sequence in a few hours.
PCR Kits for Food Microbiology
There are two broad types of detection method:
End-point PCR detection takes place, as the name suggests, when the amplification process is complete. Typically, agarose gel electrophoresis, followed by staining with fluorescent ethidium bromide is used to detect the amplified DNA fragments. This method is time consuming and not sensitive enough to measure the accumulated DNA copies accurately, so can only give a qualitative result.
Most food testing applications employ a method known as real-time PCR detection – combining the amplification and detection stages of the process so that amplification is monitored continuously during the exponential phase. Real-time detection is more accurate and the result can also be quantified.
Real-time PCR detection methods The simplest method is to use intercalating fluorescent dyes, such as SYBR Green. These fluoresce only when bound to double-stranded DNA and the increase in fluorescence can be measured at each cycle. Unfortunately, these dyes bind to all double-stranded DNA present, including any non-specific PCR products. This makes it difficult to quantify the result accurately. The problem can be overcome to some extent, but only by adding an extra stage at the end of the PCR process.
A second, more accurate and reliable method is to use fluorescent reporter probes. This method utilises an additional primer, the probe, which also binds specifically to the target DNA sequence during annealing. Probes have a fluorescent reporter dye at one end and a quencher dye, which inhibits fluorescence, at the other. During the extension stage the probe is broken apart by the DNA-polymerase and begins to fluoresce more strongly. The fluorescence emitted can be measured at each cycle and increases in proportion to the number of target sequence copies produced. To quantify the assay, the cycle at which the fluorescence intensity rises above the background level is recorded for each test sample and for a set of standards run at the same time. A standard curve can then be constructed. The amount of target DNA present in the sample can be calculated from the standard curve. Since the probe only binds specifically to the target DNA, non-specific PCR products are not detected.
Many of the commercially available PCR-based food microbiology applications use this type of detection. Probe-based detection also allows for more than one target DNA sequence to be assayed in the same sample, by using specific probes equipped with different coloured dyes. For example, some Campylobacter detection kits allow for several species of the pathogen to be detected and quantified in the same sample.
Commercial real-time PCR assays for food microbiology applications generally employ a high degree of automation to minimise the number of operations involved and reduce the risk of contamination. The reaction usually takes place inside a combined thermocycler/fluorescence detection instrument and uses pre-prepared reagents, often in dried tablet form, and validated controls. Typically, identical run conditions are used for all pathogens so that several assays can be run simultaneously. The thermocycling and detection processes are controlled by dedicated software that also calculates and interprets the results.
Typical Laboratory Work Flow A typical pathogen detection assay is performed as follows.
- Stage 1. Sample enrichment 25g of sample added to 225ml of enrichment broth and incubated overnight. Enrichment times can be as little as 8 hours but 16-24 hours is more normal.
- Stage 2. Sample preparation Collect enriched samples and lyse cells to extract target DNA. Add extracted DNA to PCR tubes/wells containing PCR assay reagents. Time 1-3 hours
- Stage 3. DNA amplification and analysis Place samples in automated thermocycler/analyser and start PCR. Dedicated software manages cycling and detection and calculates results. Time 2-3 hours
Total time for assay normally 20-30 hours but can be as little as 12 hours depending on the assay.
Benefits and Costs
The main advantage for PCR systems over other methods is in time saving, both in the total time from sampling to result and in the technician time needed to set up and run the assay. For example, a typical immunoassay-based pathogen detection method will often require a secondary enrichment stage before low numbers of cells can be detected and can take up to 48 hours to achieve a clear result. The high degree of automation built into PCR systems means that staff time and training is effectively minimised.
PCR has also been shown to have a very high specificity and generally requires fewer repeat tests than most other rapid methods, leading to further time saving. Many commercially available kits have also achieved AFNOR and/or AOAC validation.
PCR systems are flexible, allowing for several pathogens to be assayed in a single run (multiplex PCR). Real-time PCR assays can also be made quantitative without adding additional stages or operations to the assay.
Costs Capital costs for automated PCR systems are relatively high compared to those for some other rapid methods.Consumables costs are also high by comparison to rapid culture based techniques. However, there are considerable cost benefits in terms of reduced technician time and training. There is a clear cost benefit in rapid test results allowing faster HACCP verification and positive release of finished food products. A recent study carried out at the German Federal Institute for Risk Assessment (BfR) concluded that, taking all costs into account, real-time PCR could be significantly less expensive than conventional ISO culture methods for the enumeration of salmonellae in food samples.
Future developments Real time PCR systems have sufficient flexibility to allow the rapid development of new assays for emerging pathogens and for specific spoilage organisms. The facility to conduct tests for more than one gene in the same sample well is also likely to be further exploited in the future. This has the potential to reduce costs by allowing more efficient use of instrument capacity and reagents.
Get the latest updates in Rapid Microbiological Test Methods sent to your email?